How It Works — What is QPI? (Quantitative Phase Imaging)
Live cells are notoriously difficult to observe under a microscope due to their high water content and lack of natural pigmentation.
Fluorescence imaging provides improved contrast and can be used for quantitative measurements. Contrast agents are inserted into the cell (dyes, nanoparticles) or induced via genetic mutation.
The agents attach to specific cell structures (i.e. labeling) and specialized light highlights them. The excitation/emission are time limited (photobleaching), can reduce cell viability and stress the cell physiology (photoxicity).
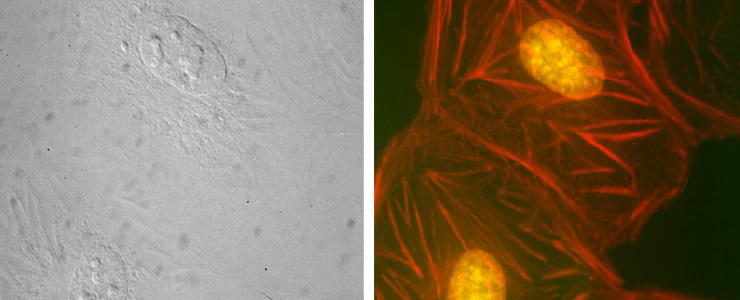
Phase Imaging with Computational Specificity (CellVista AI).
The basic principle of QPI relies on two beams of light: a portion of the light is scattered by the specimen (sample beam) and carries the information, while the second is not (reference beam). Contrast is generated from local optical path length changes (OPL) or phase shift between the beams caused by subtle variations in thickness and refractive index of the sample. QPI methods generate a quantitative map of local phase shifts in the sample.
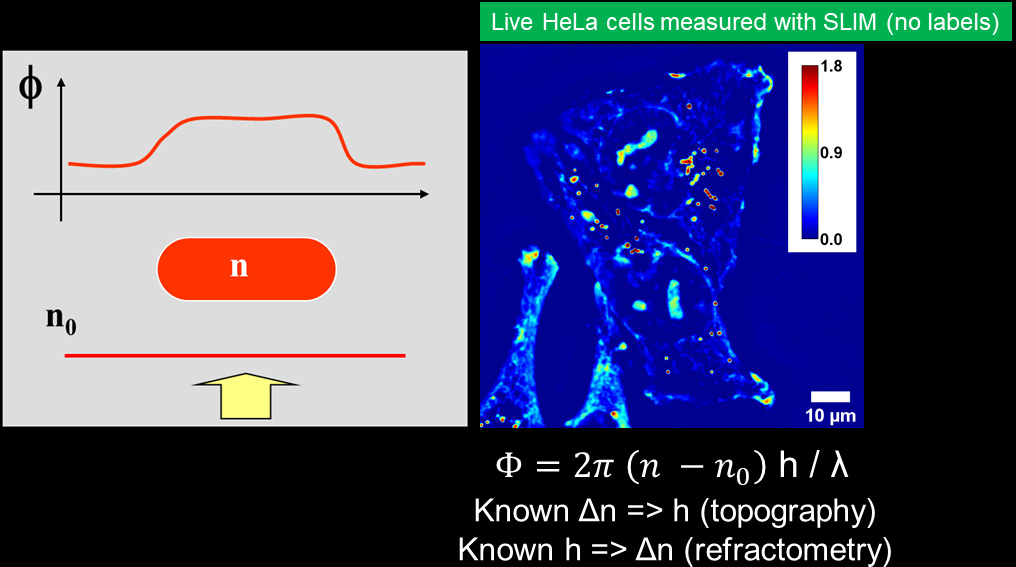
measure quantitatively
the phase shifts.
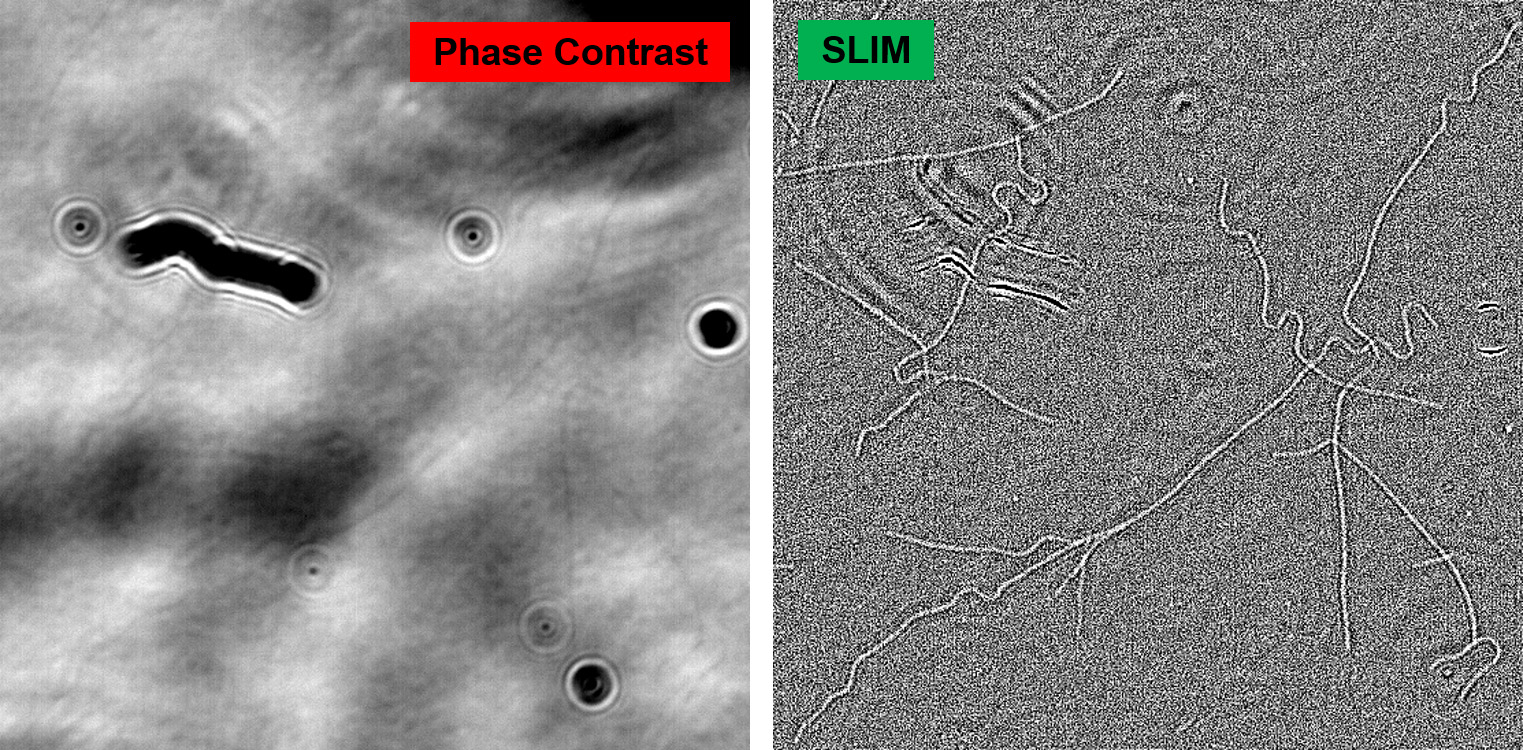
Used with permission from Kandel et al, ACS Nano 2016
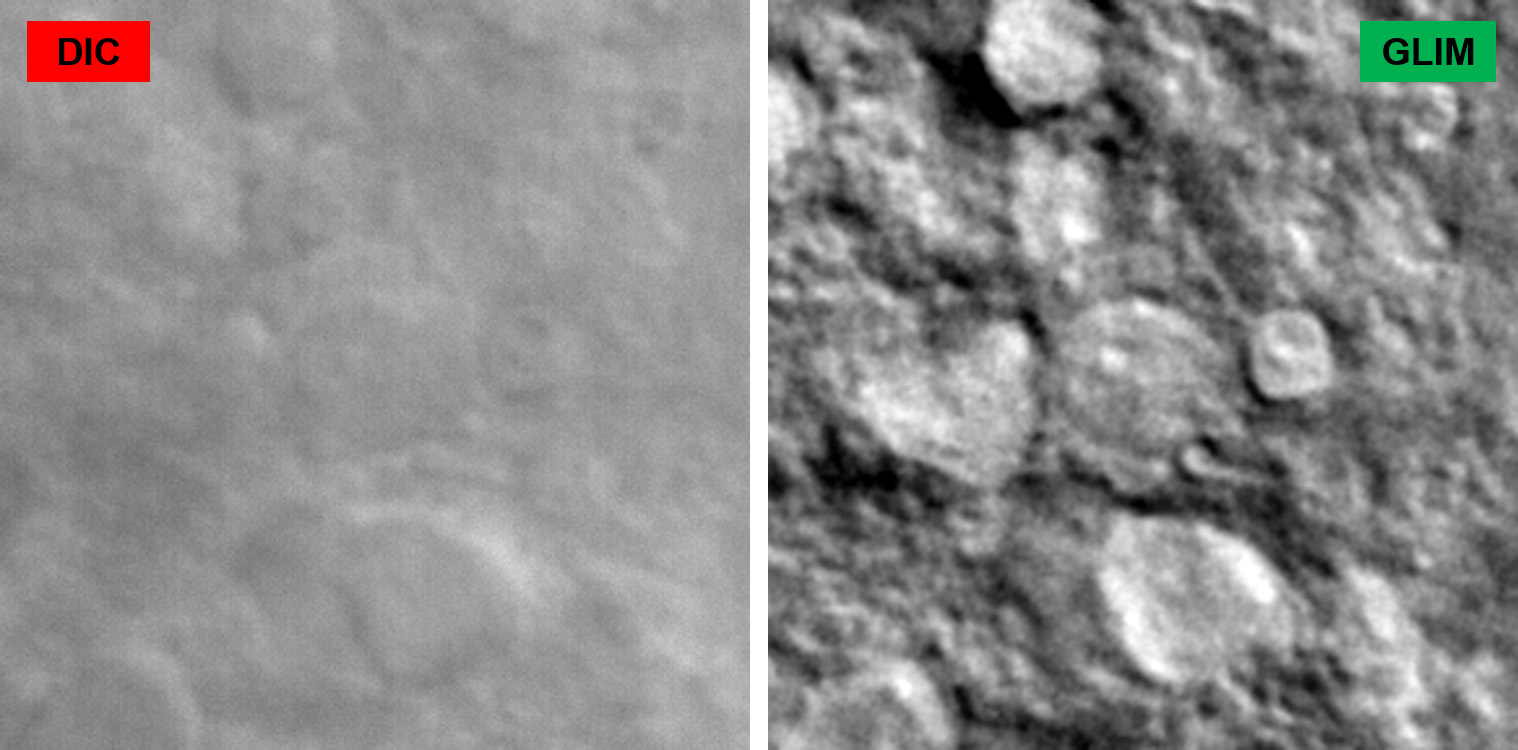
Used with permission from Kouzehgarani et al, Cells. 2022
Phi Optics combines phase imaging with low-coherence interferometry and holography in a common-path geometry. Phase imaging microscopes (phase contrast, DIC, diffraction phase) employ regular white light (low-coherence) and provide 2 illumination beams. The beams pass through the same optical elements from sample plane to the camera plane. The beams superposition is measured in every pixel (common-path interferometry) and provides high signal to noise ratio (nanometer phase sensitivity). The direct quantitative phase map of the specimen (holography) is then recovered: pixel intensity in a QPI image is a local measurement of the phase shift (in radians). This phase map can be converted to local thickness, refractive index and dry mass maps of the specimen.
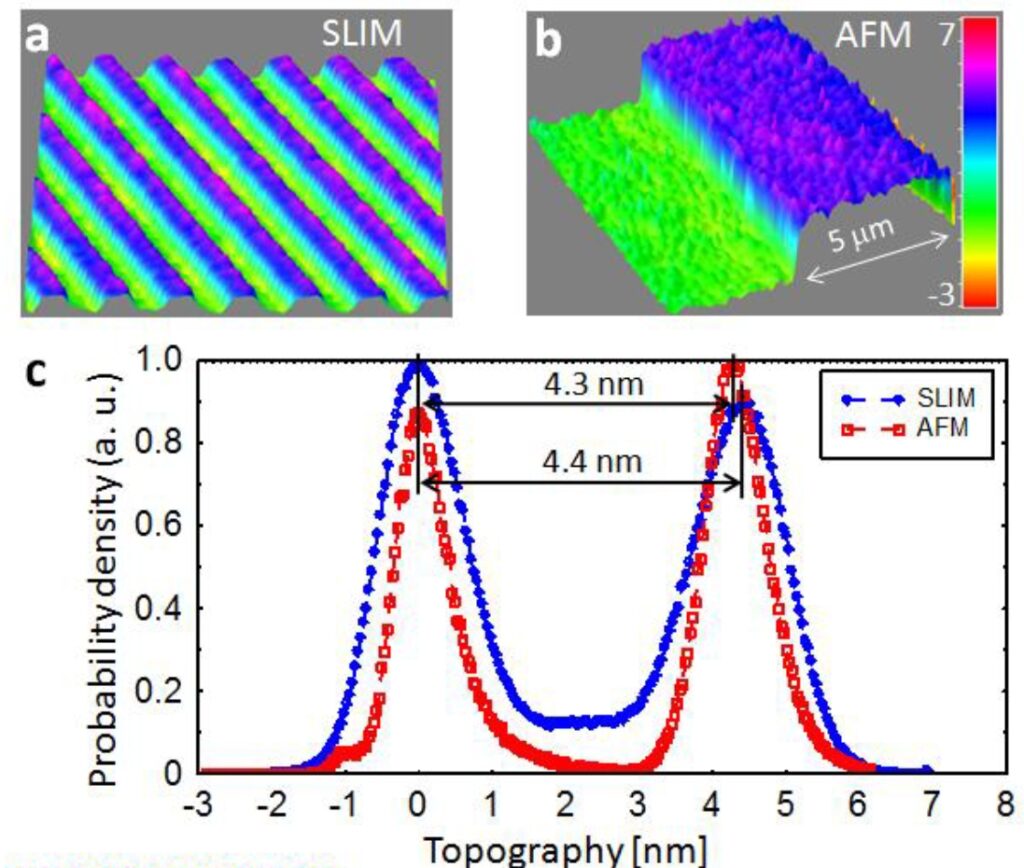
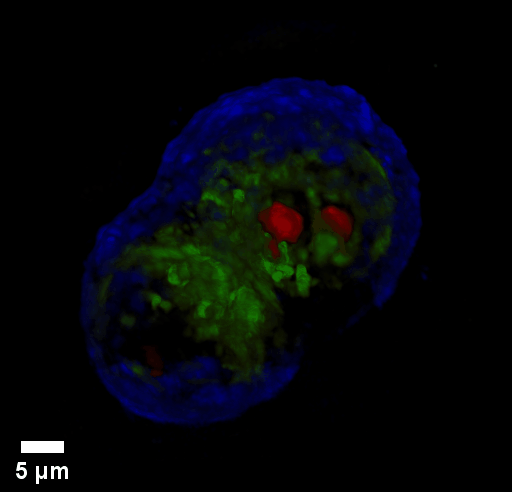
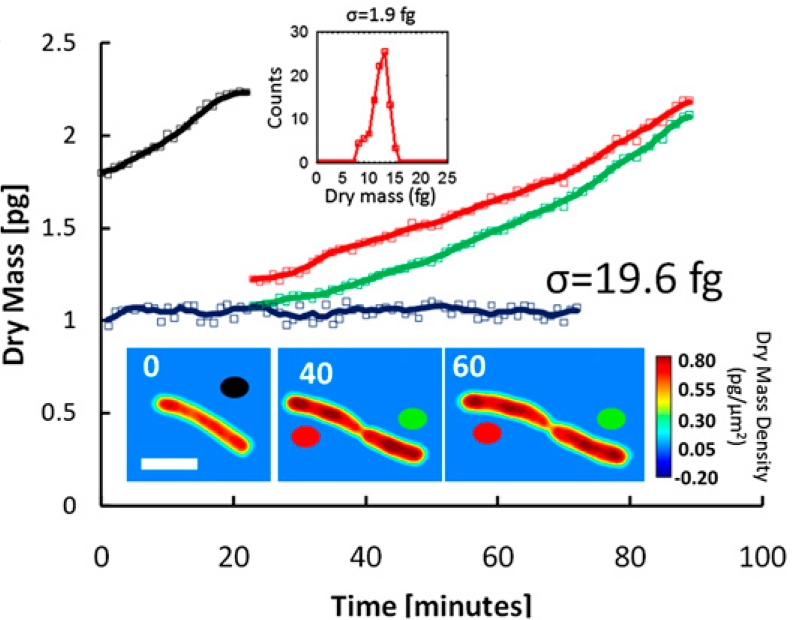
The use of white light illumination provides a few advantages to CellVista SLIM™ & CellVista GLIM™ techniques that makes them a perfect companion for fluorescence imaging. It avoids the speckles that plague laser-based illumination QPI techniques and provides a low noise floor therefore a flat measurement background. Combined with common-path interferometry, Phi Optics imparts nanometer phase sensitivity for minute features: lipid nanoparticles, microtubules, viral capsids aggregates, mycoplasma contamination.
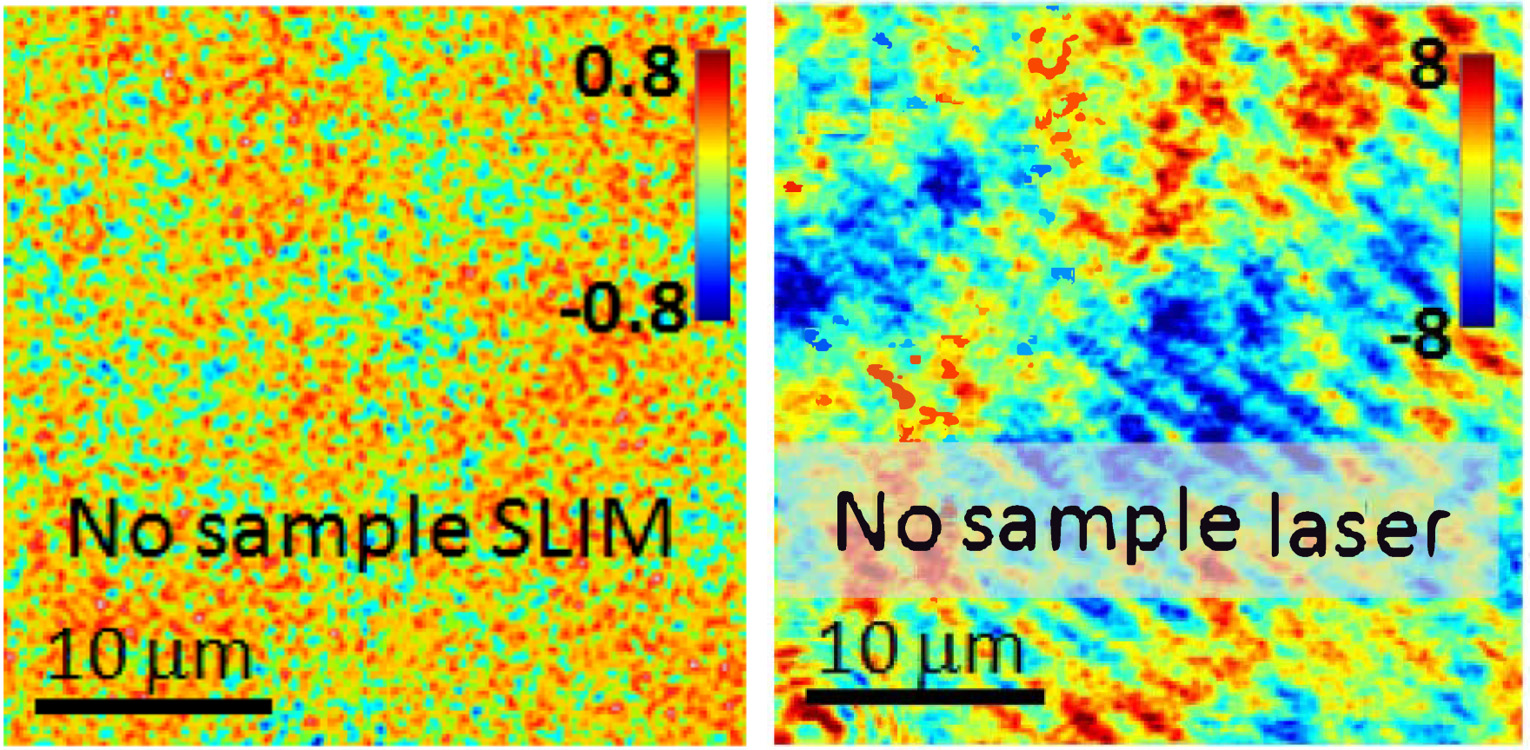
Phase measurement noise level in a background image (i.e. no sample) for SLIM vs. laser-based QPI. Color bars in nanometers (modified with permission from Z. Wang et al, Opt. Exp., (2011))
Coherence gating due to the white light illumination source improves the optical sectioning afforded by high NA objectives for accurate 3D tomography.
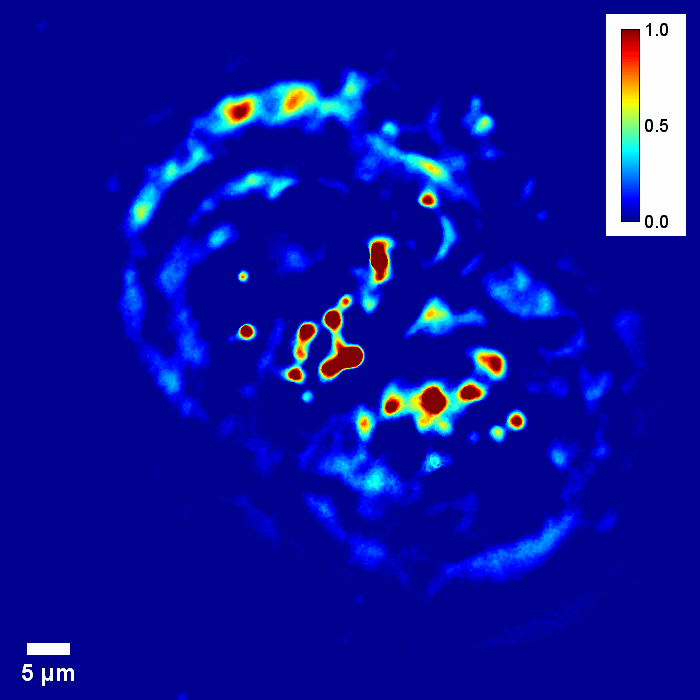
Through focus SLIM Z-stack of HT-29 live cell. Color bar is phase in radians (with permission from T. Kim, R. Zhou et al)
Through focus GLIM Z-stack of HEPG2 spheroid.
Low-power density (compared to laser sources) of the white light illumination allows continuous, long term imaging (from seconds to days) without photobleaching and phototxicity. CellVista SLIM™ and CellVista GLIM™ can measure various assays (proliferation, cytotoxicity, immune cell killing, and so on) while co-localized fluorescence is used sparsely for dynamic segmentation of regions of interest (ROI).
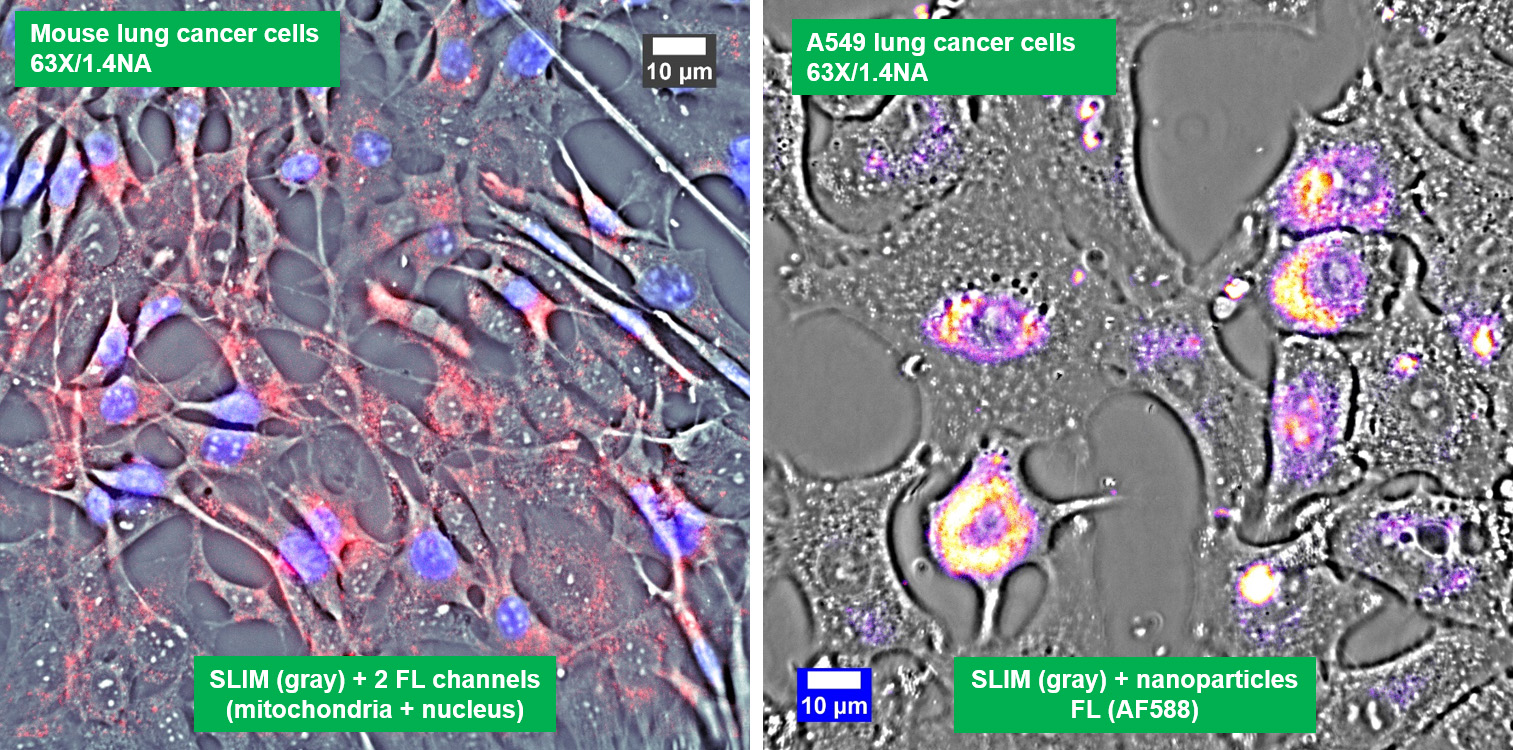
Co-localized quantitative phase and fluorescence channels
Phi Optics QPI Modules
CellVista SLIM™ & CellVista GLIM™
CellVista SLIM™ & CellVista GLIM™ seamlessly upgrade commercial microscopes (Zeiss, Nikon, Leica, Olympus) with any magnification available (immersion or dry). Either module connects to the imaging port of a microscope. Samples load into standard holders (glass slides, single, multi-well plates) and fields of view are limited only by the microscope stage movement. Phi Optics modules use the same camera for all channels of imaging from the microscope therefore insuring seamless overlays. High end sCMOS cameras provide high sensitivity fluorescence co-localization. Any fluorescence channels present on the microscope can be used for imaging.
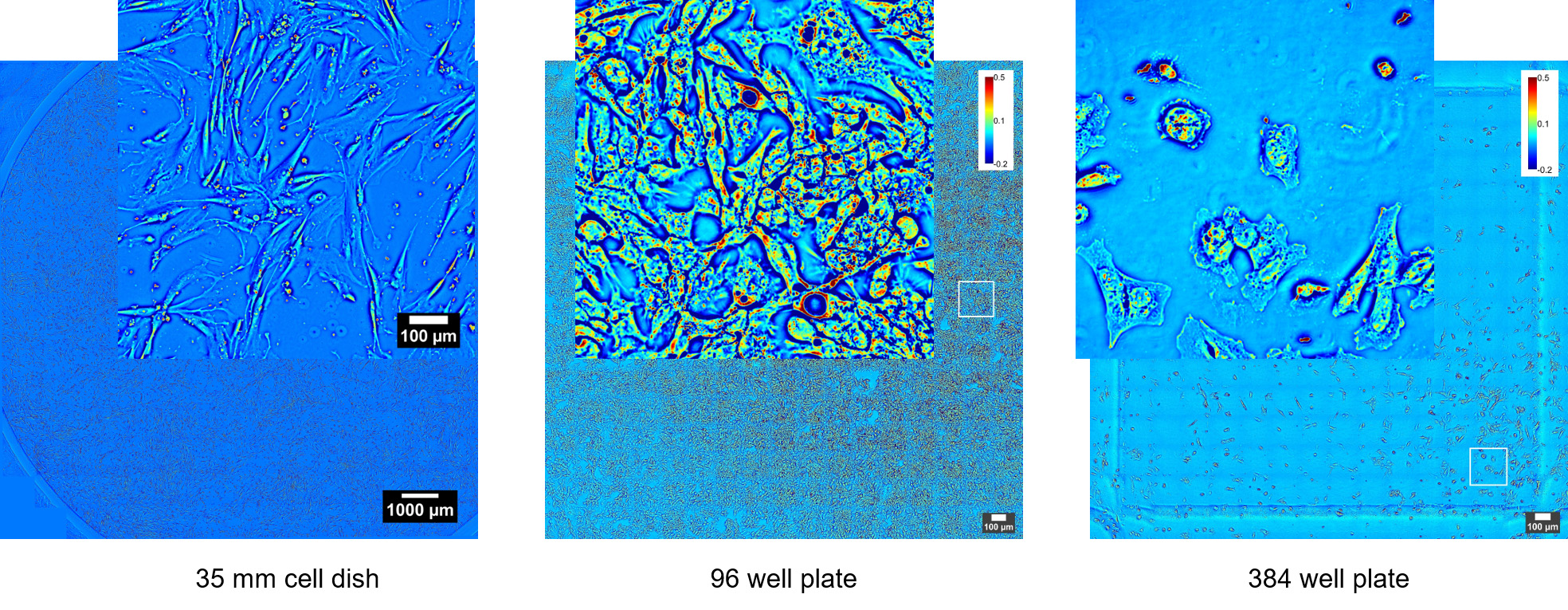
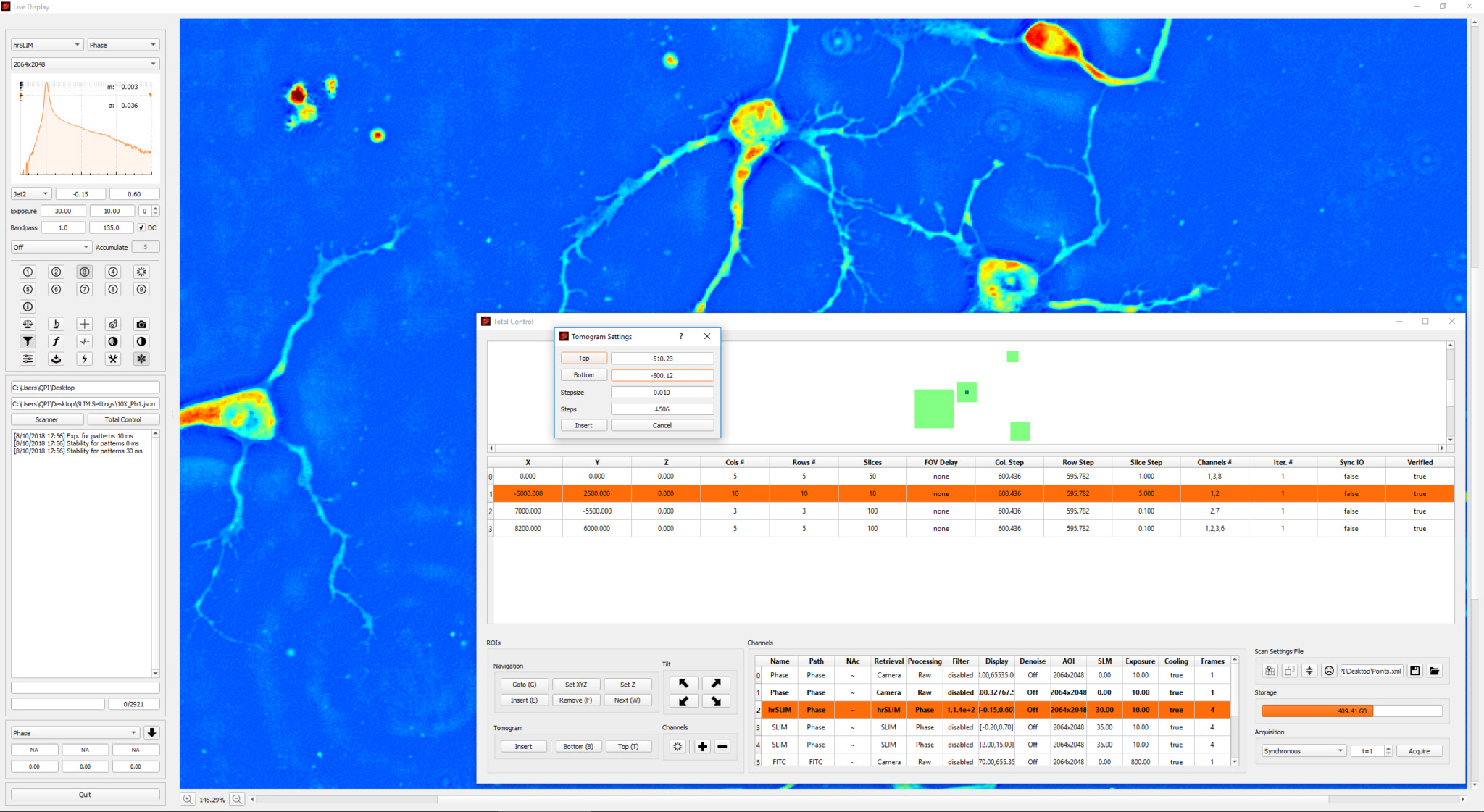
Phi Optics CellVista™ acquisition software platform can control the microscope frame motorization for programmed 4D (3D and time lapse) multichannel (QPI and fluorescence) monitoring of live and fixed cultures.
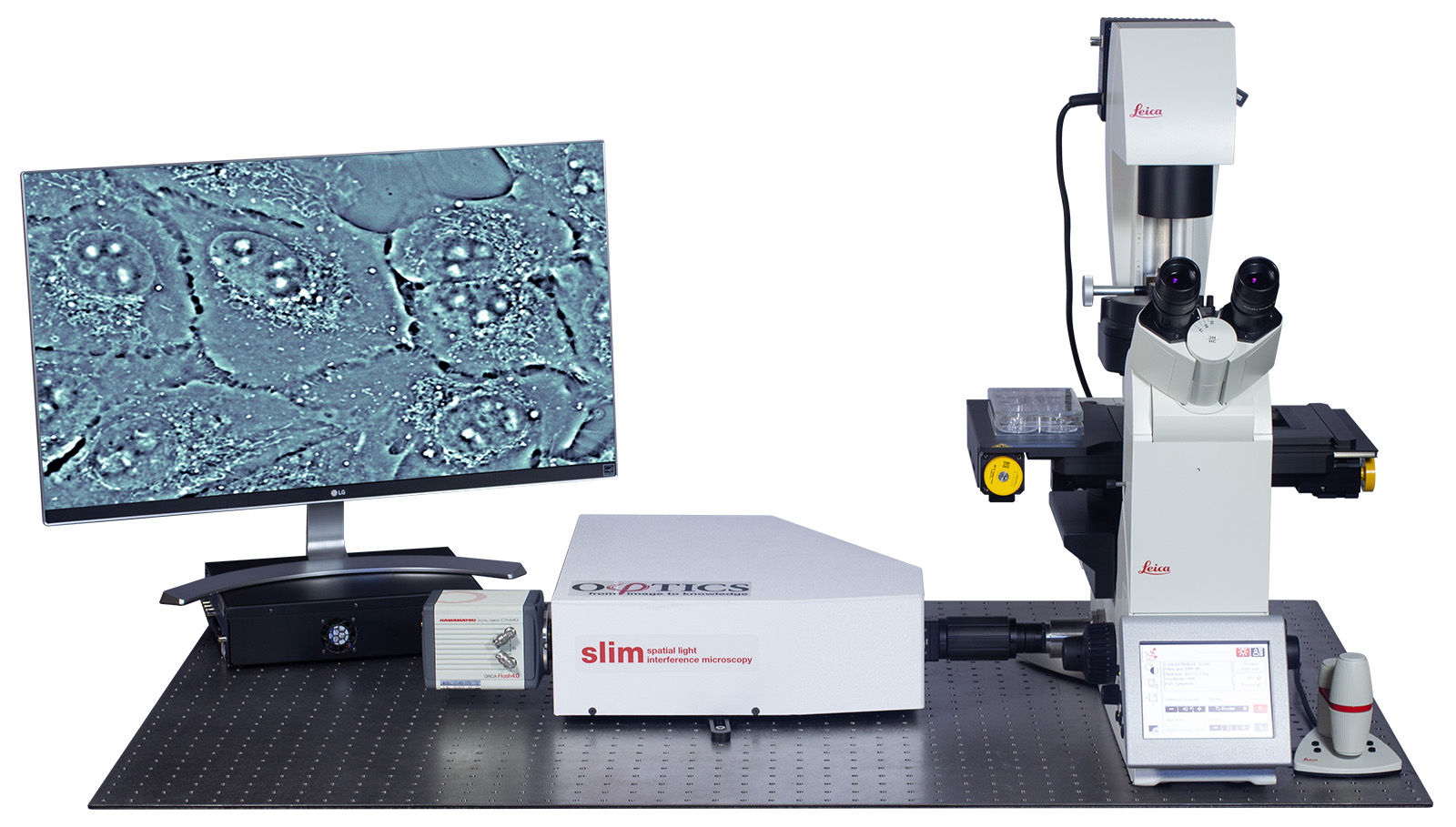
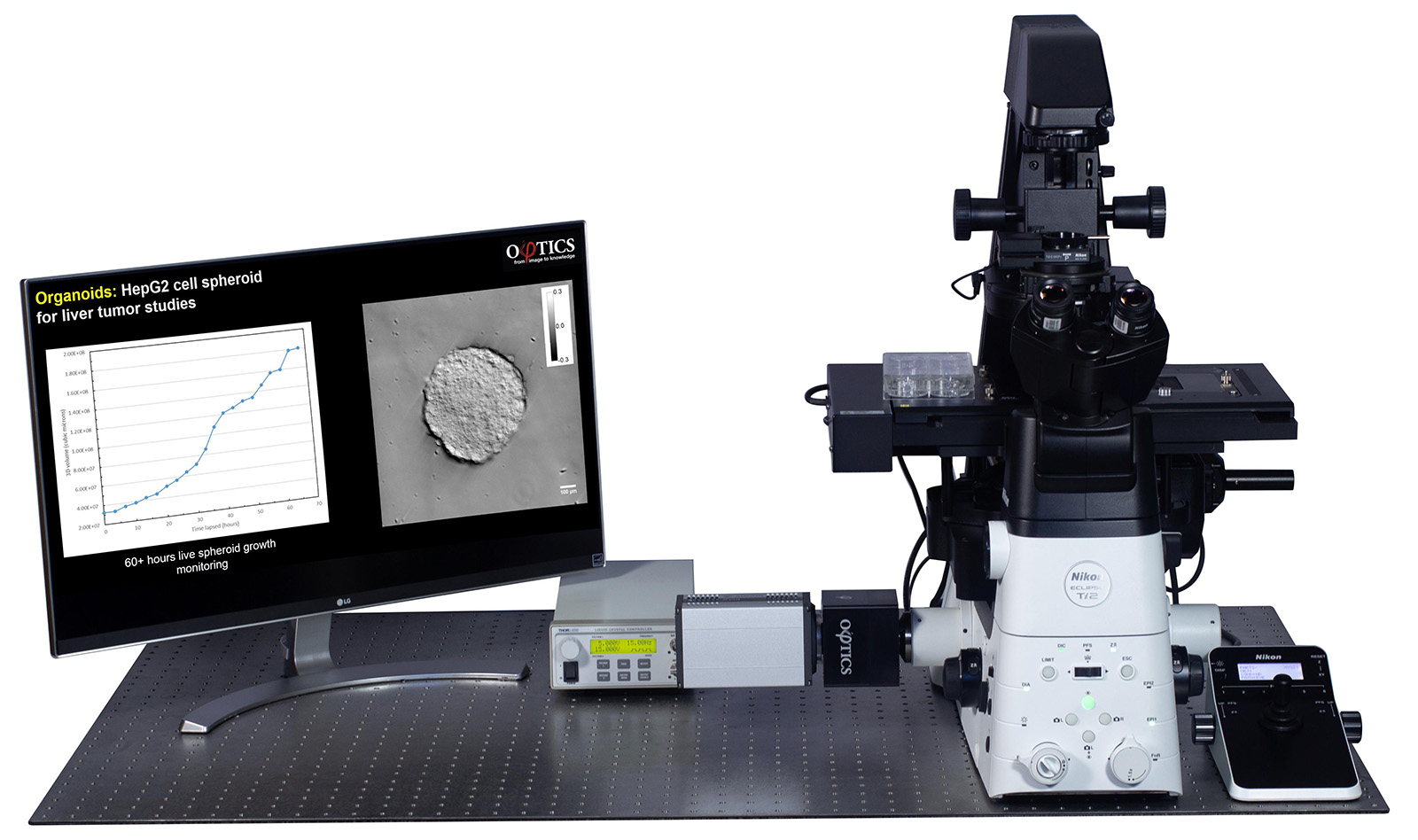
CellVista GLIM™ module attached to a Nikon Ti2 microscope (Microscope sold separately)
See the latest publications here. For more information about customization don’t hesitate to CONTACT US!
References:
The power of imaging with phase (2017)
Spatial light interference microscopy (2011)
Gradient light interference microscopy (2017)
Whitelight diffraction phase microscopy (2014)
Quantitative Microscopy/Drug Discovery: BioOptics World (2018)
Quantitative phase imaging in biomedicine, Nature Photonics 12,(2018)
Patents: CellVista SLIM™ (US Patents 8,184,298, 8,520,213, 9,052,180, and 9,404,857) CellVista GLIM™ (US Patent 10,132,609)